Genome decay over millions of years
Behind the Paper: Genome Evolution in Bacteria Isolated from Million-Year-Old Subseafloor Sediment
A behind the scenes look at Genome Evolution in Bacteria Isolated from Million-Year-Old Subseafloor Sediment
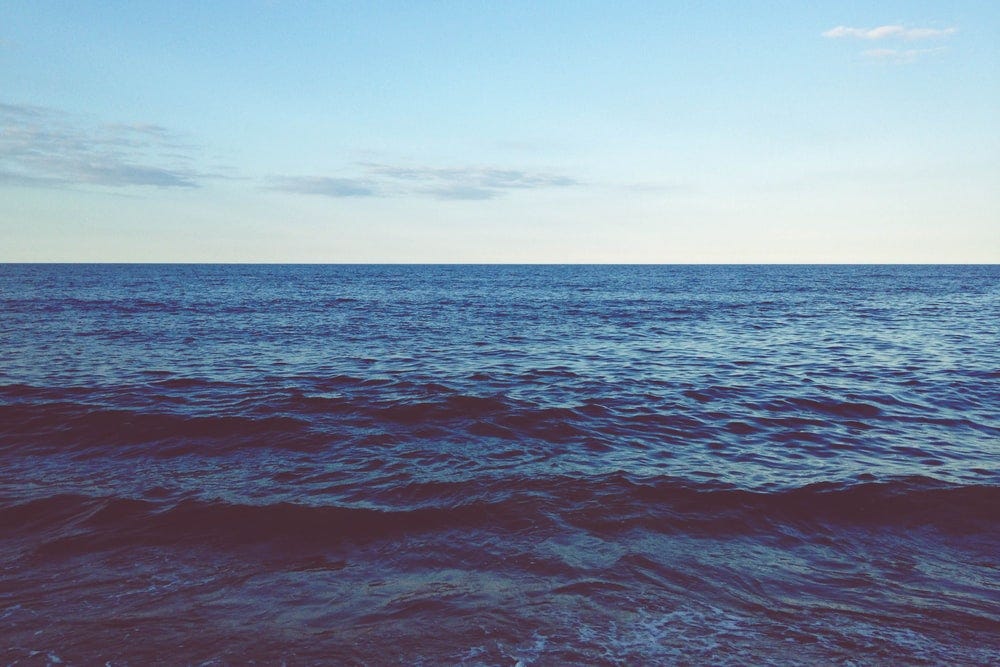
How do microbial genomes evolve in cells that are perpetually starved?
Microbes in the dark ocean and deep-sea sediments are key mediators of global nutrient cycles and long-term carbon sequestration. Yet, most of these microbes are not actively dividing due to the limited supply of energy-yielding growth substrates, such that in situ growth rates approach zero. Whether these microbes can evolve at these excruciatingly slow growth rates and what that evolution looks like has remained elusive. Although “long-term” laboratory studies (to humans—up to 10’s of years) of energy-limited microbes have provided clues as to the evolutionary forces at play in low-energy environments, most researchers find it difficult to fund million-year-long projects. There is simply no substitute for millions of years of perpetual starvation.
The project started in 2014 when Bill Orsi and Steve D’Hondt successfully cored deep sea abyssal clay (sediments) from a water depth of 6,000 meters in the North Atlantic Ocean. It’s hard to core seafloor sediments in deep water. Imagine trying to land a 30 m long coring device on the seafloor at a vertical distance of 6,000 m from the ship and not over-penetrating the seafloor (or missing it completely). It’s the ultimate confluence of experience with a bit of luck. Steve D’Hondt and his research team are probably the best folks in the world at coring seafloor sediments in deep water.
The purpose of the cruise was to investigate how life survives in deep subseafloor sediments (up to 30 m below seafloor) with plenty of oxygen, but few food sources for carbon-eating microbes. This is a rather unique setting because subsurface sediments often contain little to no oxygen. Though there may be ample energy in the form of organic carbon in these oxygen-depleted sediments, the microbes survive by breathing compounds other than oxygen—a less energetically favorable mode of existence.
The rate at which sediments are deposited in the open ocean is extremely slow because of restricted algal productivity in the overlying waters (about 1 meter of sediment per million years). This causes the deposited organic matter to sit on the surface of the seafloor for a long time before it is buried. As it sits on the surface, carbon-eating microbes slowly decompose the organic matter until it is nearly all consumed. Thus, the carbon-rich sediments are near the seafloor surface and as time passes, and new sediment is deposited, the underlying sediments below the seafloor surface become extremely depleted in organic matter concentrations. The microbes in these extremely low carbon sediments cheat death by living near the energetic limits of life where they have access to just enough food to not die, but not enough to reproduce (or at least reproduce on human timescales).
Bill set up a long term (1.5 year) stable isotope probing experiment with 18O-labeled water to look at the metabolic activity of the subseafloor bacteria from sediments that were deposited 3 and 6 million years ago. In these experiments, 18O-labeled water is added to sediments in bottles. Microbes that are actively dividing incorporate the 18O-labeled water into their DNA, making it more dense. This denser DNA can be separated from lighter DNA by high-speed centrifugation and sequenced to identify microbes that are actively synthesizing DNA in a sample. The results of this experiment showed that these oxygenated, low carbon sediments were anything but dead! Many of the microbes present in the sediment became labeled with the 18O-labeled water, demonstrating that the microbes detected in the sediment column are indeed living (Vuillemin et al. 2019). Further, these results indicated that at least some of the DNA in these sediments originated from living cells and is not relic DNA preserved from the ancient past (Carini et al. 2016).
At the end of the 1.5-year 18O-labeled water incubation, Bill decided to try and cultivate bacteria from the slurry incubations. The cultivation strategy was simple. Bill asked himself “what do subseafloor bacteria like to eat?” Most evidence indicates that subseafloor cells generally feast upon dead cells, sort of like zombie bacteria. So, he decided to make a growth medium for the bacteria that is based on dead cells: yeast extract (e.g., dead yeast cells). After plating the sediment slurries from the stable isotope incubation onto petri dishes containing yeast extract, colonies appeared. These were isolated into pure cultures and frozen in glycerol.
Bill & Paul had dinner at the 2019 ASM Microbe meeting in San Francisco (at an amazing dim sum restaurant in Chinatown that neither of us can recall the name of) and chatted about the cultures and how the evolution of the bacteria could be studied. At the time, the data was new and the scientific questions were not well-defined. Bill’s lab group had draft genomes of the strains and were in the beginning stages of analyzing them. Bill had contacted Jesse Shapiro about the potential role recombination might play in the evolution of genomes from the subsurface isolates. Jesse suggested to calculate rates of recombination and mutation between the different strains. This clearly showed that the subseafloor bacteria had extremely low recombination rates, relative to closely related surface world strains of bacteria.
Fast forward to April 2020. What started as a casual zoom meeting for Bill and Paul to catch up as friends to discuss how COVID-19 had affected us, evolved into the final paper. Initially, Bill wanted some advice on how to set up growth experiments for the subsurface isolates. As we started discussing the genomic data it became apparent that something interesting was going on. Low recombination rates in environmental bacteria is relatively uncommon and stood out as unusual. However, low recombination rates are common in microbial symbionts of insects that undergo a very specialized type of genome evolution driven by genetic drift. Because recombination is limited in these symbionts, they are clonal populations and have small effective population sizes. The effects of clonality are that genomes are more likely to pass deleterious mutations on to the next generation through genetic drift. In the absence of recombination, this leads to a ratcheting like mechanism where genomes slowly decay into a collection of damaged genes (pseudogenes), which are eventually lost. Could a similar type of evolution be occurring in the subseafloor isolates? We investigated whether there were signs of this type of “symbiont-like” evolution in the subseafloor genomes and indeed found that a higher proportion of damaged genes in the genomes of the subseafloor isolates and evidence of a relaxed selection on some types of genes.
However, the deep biosphere strains did not exhibit the extreme genome decay and genome size reduction that is a hallmark of insect symbionts genomes. But maybe that’s to be expected? Insect symbionts reproduce much faster (on the order of the insect’s lifespan), and within a nutrient-rich environment that makes many genes expendable. Moreover, there are severe symbiont population bottlenecks with each insect generation that ratchet genome decay relatively rapidly forward in time. In contrast, subseafloor bacteria are initially deposited to the seafloor and are buried over millions of years. As they are buried, available food is consumed to growth-limiting levels causing their growth rate to continually slow over time, extending the generation time to 10-‘s to 1000’s of years or more. Since acquired mutations or gene decay are passed to the next generation through cell division, the accumulation of these mutations and pseudogenes may appear much slower from a human perspective than in insect populations—because the generation time is drastically slower. Thus, we might not expect to see the extreme level of genome decay in the deep-sea bacteria that is observed in insect symbiont genomes simply because insufficient time has passed to observe them.
The coolest part of this story is that we’ve got several cultures from microbes that normally live in the deep sea! The experiments that we can conduct and the questions that we can ask are endless! Already, we’ve identified some interesting gene suites in these microbes that might help them persist in super-low-nutrient environments. There is also evidence that transposons and phage might play a role in the deep biosphere. Many exiting things to come!
If you are enjoying Un-Cultured, please like, share, or leave a comment below.