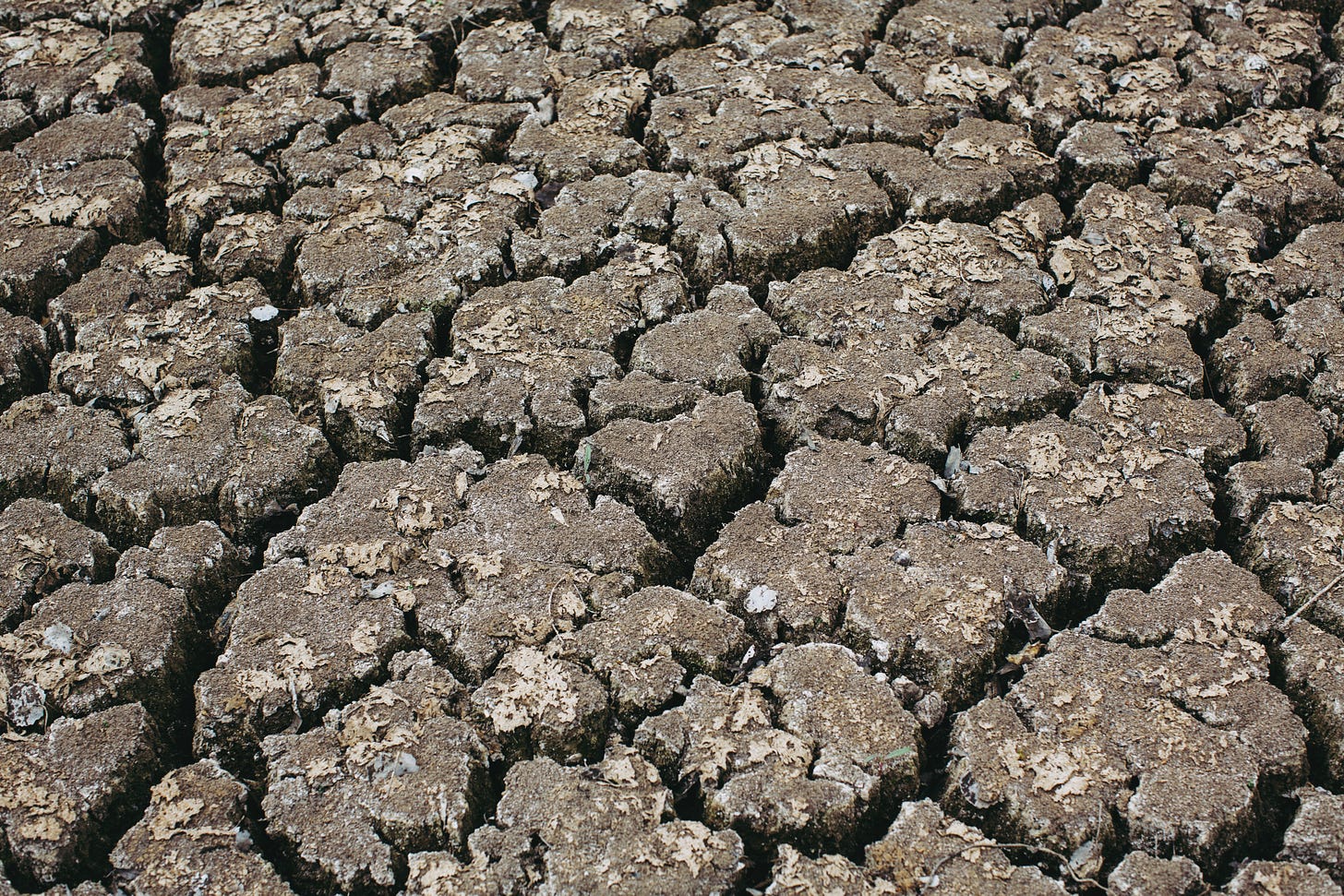
Recently, in Nature Microbiology, Sean Bay and colleagues discovered that a large portion of soil microbes—mostly heterotrophs—use trace gasses as supplemental energy sources across diverse soil types (Bay, et al 2021).
The finding that trace gas oxidization is so widespread in soil and occurs across the bacterial tree of life is new and exciting. Trace gasses are ubiquitous in the atmosphere and are pervasive in soil where they are produced and consumed by microbes. As outlined in the accompanying News & Views article, accounting for trace gas metabolism may affect the way we interpret carbon use efficiency (CUE) in soil ecosystems (Carini, 2021).
Despite the interesting finding that trace gas oxidation is so widespread, I found myself drawn to a more subtle aspect of the paper: the ubiquity and abundance of formate oxidation marker genes (from their Figure 1). Formate (CHOO−) is a low molecular weight volatile organic acid that is present and sometimes abundant in soils as a constituent of plant root exudate, a product of microbial metabolism, and the result of several abiotic reactions. Like trace gasses, soils are implicated in the balance of atmospheric formate, though constraining these fluxes has been challenging (Mielnik, et al. 2018).
Aerobic degradation of formate is primarily performed by microbes coding for the formate dehydrogenase enzyme. Formate dehydrogenase oxidizes formate to carbon dioxide and captures the released energy in the form of electrons to use for respiration. Some microbes can fix the resulting carbon dioxide into new biomass through autotrophic carbon fixation pathways. Thus, much like the oxidation of trace gasses, aerobic soil microbes may oxidize volatile formate for use as an auxiliary energy source.
King and Weber proposed the concept of ‘carboxydovory’ to describe the metabolism of microbes that oxidize carbon monoxide to carbon dioxide but cannot use carbon monoxide as a sole carbon source (King & Weber, 2007). They speculated that carbon monoxide oxidation may be used as a supplemental energy source or to fuel mixotrophic growth. The new paper by Bay and colleagues show that carboxydovory is indeed widespread in soils.
Sun et al. (2011), extended the carboxydovory concept to organic compounds—specifically, C1 methylated compounds or methyl groups derived from organic matter—to coin the term “methylovory.” Methylovory in this context describes the oxidation of one-carbon compounds (or methyl or formyl groups) extracted from a suite of simple-to-complex molecules as a source of energy, but not a source of carbon for new biomass production (Sun, et al. 2011). That is, methylovores have a partially bifurcated metabolism in which the respiration of organic C1 compounds is decoupled from the assimilation of organic carbon for new biomass production.
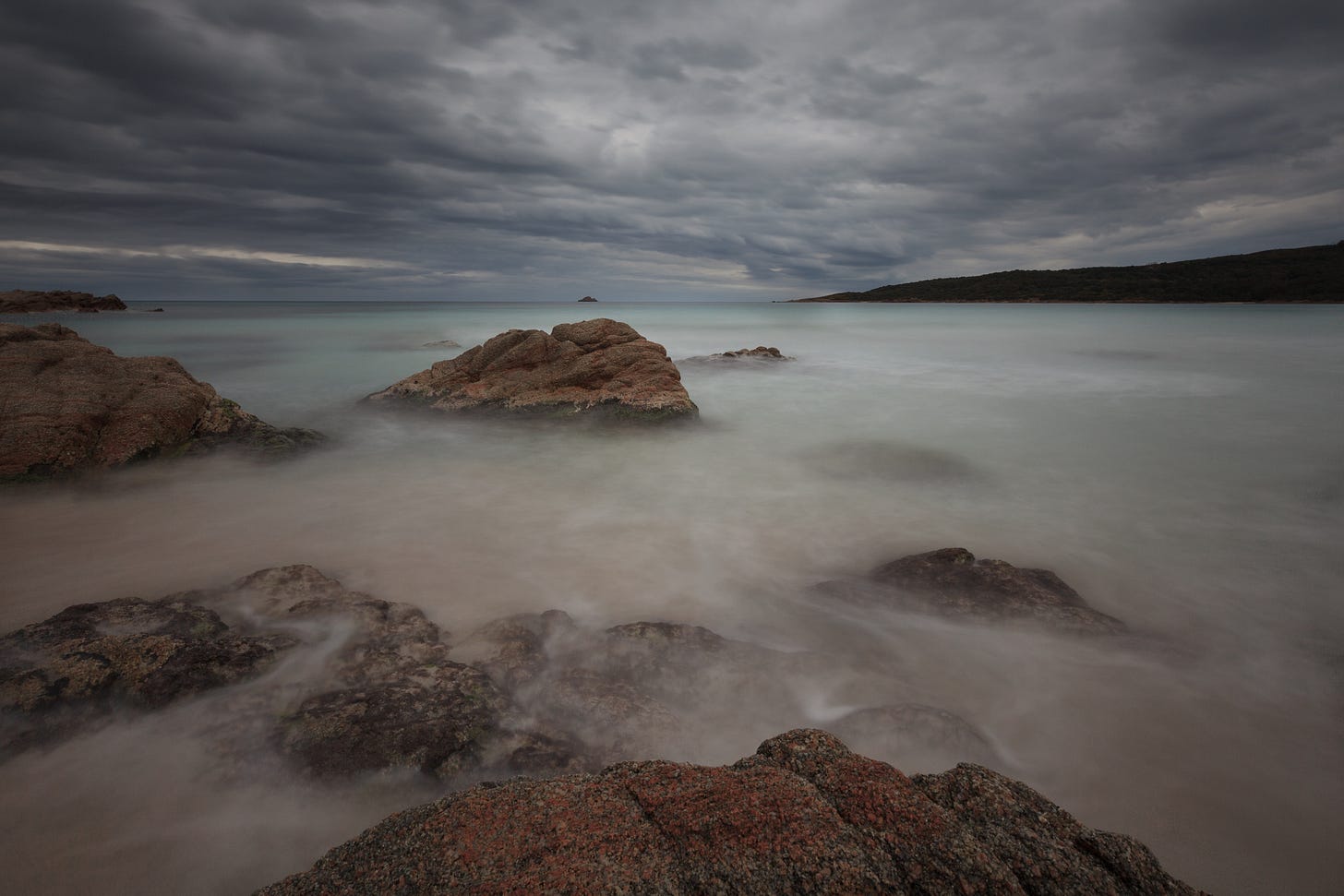
In addition to showing the widespread nature of trace gas metabolism, Bay’s work shows that the use of formate or formyl groups derived from organic compounds appears to be a widespread form of methylovory in soil. While free formate is ubiquitous in soil, we know very little about the abundance and ubiquity of formyl groups bound to soil organic matter. Deformylase and formamidase enzymes catalyze the cleavage of formate from diverse organic compounds. Cleaved formyl groups from soil organic matter may be an important energy source for microbes that code for both formate-cleaving enzymes and formate dehydrogenase. Thus, formylovory—the use of formate or formyl groups derived from soil organic matter as an energy source—is potentially a widespread activity in soil microbiomes.
The way that microbes extract energy from compounds found in soil vapors is reminiscent of the discovery that bacterial rhodopsins are prevalent and active in marine microbes. Bacterial rhodopsins are proteins found in surface-dwelling marine heterotrophs that allow cells to derive energy from sunlight (Béjà, et al. 2000). This ‘passive’ energy generating mechanism confers several benefits for marine bacterioplankton in sunlit waters, including increased fitness during long-term starvation (Gómez-Consarnau, et al. 2010), sustained nutrient transport in carbon-limited conditions (Steindler, et al. 2011), and growth stimulation (Gómez-Consarnau, et al. 2007). In part, these benefits arise because rhodopsins subsidizes part of the cellular energy requirement that would otherwise come from organic carbon, thus allowing a larger fraction of consumed carbon to be allocated toward growth or cell repair.
Although the oxidation of gaseous or volatile compounds and rhodopsin-mediated energy generation are biologically distinct processes, soil gasses and volatile organic compounds found in soil vapors are an ever-present diffuse source of energy in soil, much like sunlight is omnipresent in the surface ocean. Future studies will undoubtedly reveal that, like proteorhodopsin, the compounds found in soil vapors offer a multitude of physiological and survival benefits for soil heterotrophs.